Data Streams: How wireless technology is transforming river science in the Rockies
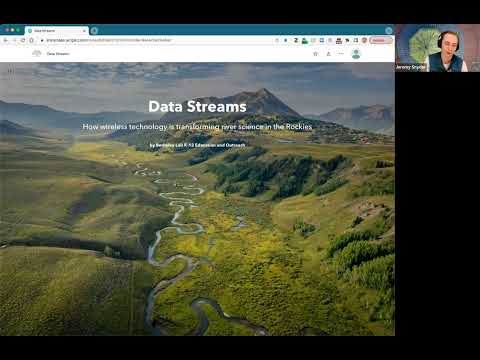
Well, in the in the interest of time, I think I'm going to get starte at the very least with just introducing myself and kind of some of the basics of what we're here for today. My name is Jeremy Snyder. I had the privilege of kind of taking the lead on this project helping encapsulate some of the really interesting research that Berkeley Lab is doing here in the Colorado Rockies on River Science.
And Berkeley Lab is a Department of Energy National Laboratory, which is kind of a public research institution. It's one of the biggest in the country. And since 1931, they've been hand tackling teams or sorry, tackling public research interests and have been awarded 16 Nobel Prizes and have been responsible for 16 of the elements on the periodic table.
It's one of the biggest research organizations in the country, and they do some really cool work, one sliver of which we'll be discussing here on this Story Map. And I'm here today with a couple of other folks, two of whom are on the K-12 Education Outreach team who kind of sponsored and promoted this Story Map. So I'm going to have them introduce themselves starting with Faith. All right, Jeremy, thank you. Hello, everybody. And thank you for joining us this evening.
My name is Faith Dukes and I'm Director of K-12 programs here at Berkeley Lab. Hi everyone. My name is Alisa Bettale.
I am the Content & Instruction Coordinator for K-12 programs here at Berkeley Lab. Amazing. And we'll hear a little more about specifically what K-12 programs do and how they play a really awesome role in getting folks to have the opportunity to work at Berkeley Lab and get into STEM fields. But in the meantime, I'm also going to introduce two of the members of the scientific organizations whose work is actually profiled here in this story map. And I'll start with Ken Williams.
Hi, everyone. I'm Ken Williams and I am a geoscientist at Berkeley Lab. I actually was responsible for taking some of the first sample that we'll be hearing about today in the East River watershed back in May of 2014. It's been a remarkable journey between there at that point in time and where we are today. Awesome and Andrew. Sorry you have to enable my video, but hi everyone.
I'm Andrew Wiedlea. I'm a network engineer with ESNet which is the Energy Sciences Network. And we are we are very lucky to have great scientists who we can collaborate with, like Ken. Amazing. So
this story map is essentially a look into like I said, one small sliver of Berkeley Lab's research. And as a public institution, most of Berkeley Lab's research addresses large scale societal needs of some kind. And so to understand why that what this work is and why it's so important, it makes sense to start with what that need is. And at the core of it, of the need for this work is the Colorado River And the Colorado River is a fairly, you know, household name at this point. And that's because it is one of the most extensively used and fought over rivers in the world Its water supports 40 million people across the western United States, which pans out to more than one in every ten Americans.
And because it has so many mouths to feed and such an important role in really half of the country by area, it's really essential to understand and be able to manage how much water is in the river. And especially in the face of kind of increasing ratification of the western United States, ongoing drought and the future of climate change. So Berkeley Lab has been working on doing that for a long time, and that is really at the core of what this research that we're going to be hearing about today is. So in a map view, you can see this is the course that the Colorado River takes from the Colorado Rockies all the way down to the Sea of Cortez in Mexico. And you can see in the satellite image that it really dominates this kind of western half of the United States, which is by far the browner half.
It is very, you know, it's defined by its aridity, by its desert climates. It is a very dry, you know, kind of arid area. And as a result, the Colorado River really represents kind of an artery or a lifeline to a lot of the people and industries in this area. So I'm going to take a second here to ask if people can just add to the chat, whether they have heard, whether it's in the news or, you know, through books, whatever you whatever about the kind of ongoing drought and increasing drought on the Colorado River and whether that has affected you in any way that you're aware of.
Yeah. I mean, I think the headlines that most people will have seen are around Lake Powell, Lake Mead, the the kind of the famous bathtub ring of the reservoirs that are getting lower and lower. It's kind of this pervasive problem. But the impacts on, you know, people's everyday lives who don't live on Lake Mead are not always obvious. But in spite of that, pretty much everybody in the western United States is impacted by the Colorado River in some way.
Whether that's because they use electricity that's generated by these massive dams along its length, like the Glen Canyon Dam and the Hoover Dam, whether it's because they eat be beef that was ranched on its shores in Colorado, or even if they drink its water even if they're hundreds and hundreds of miles away from it, because its water gets piped across the country and aqueducts like the Central Arizona project, or if it's just because they eat lettuce in the winter, which the vast, vast majority of which is grown in California's Imperial Valley, which is irrigated with Colorado River water. So whether or not you know it, the Colorado River probably plays a role in your life and to to study it and to understand how much water is going to be in the river each year, scientists need to look to its source in the Colorado Rockies. And that's because this is the area that the Colorado River drained at its watershed or basin. So any water that falls in this area highlighted in blu will eventually flow down the hill and end up in the Colorado.
And you can see already in the satellite view that that is you know, it's a pretty dry area. But in this precipitation map, where orange and yellow represents low, very low annual precipitation and blue and green represents high precipitation, you can see that really the only areas in that watershe are here along the spine of the Rockies, which means that 80% of all the water that ends up in the river comes out of these little mountain tributaries. So if you zoom in, the Colorado River basin as a large watershed is made up of all of these kind of constituent watersheds that basically funnel into small tributaries that eventually can, you know, have a confluence with the river and add their water. These mountain watersheds are what are really important to understand. If you are to understand, to be able to predict and manage the water that ultimately ends up downstream in the Colorado River itself. And one such mountain watershed is the East River Valley in Crested Butte, Colorado.
So this is one of the best studied mountain watersheds in the world, and it has been for years and years. And scientists have been using this as kind of a model watershed to understand the mountain watersheds everywhere. And at the core of this activity and the core of the science is really this idea of modeling, which is worth taking a second to to talk about, because it's a really core scientific tool.
The idea behind scientific models is basically if you can create a really accurate digital representation of a landscape or, you know, an environment , and you can combine that with just what we know about the laws of physics, you can simulate future scenarios really accurately. And so you can say, you can predict, say what would happen if you drop a million gallons of snow in one specific part of a watershed? How that's going to flow downstream, how that's going to end up in the water, how it's going to affect ecosystems and livelihoods. And so a large part of the science and really the policymaking of river management is tied up in these models. And we have already pretty good models based on a lot of the work that has already been done in places like the East River watershed.
But as the stakes grow higher and as we face an increasingly uncertain future with climate change, it's ever more important to collect more and better data about what is actually happening on the ground so you can really increase the accuracy of those models. So this is the story of how scientists in the East River, including the folks we have on the call today, are making that leap and are entering kind of the next generation of data collection in this essential watershed that is a tributary of the Colorado River. So the solution to this problem of monitoring and understanding mountain watersheds is tied up, is really rooted in studying watersheds like the East River and the East River Valley, like I said, is one of the best studied in the world. It's dotted with these sensor stations that are kind of scattered around and collect information about how water moves through the landscape.
This is what they look like. They're really kind of glorified weather stations, but they're purpose is to track water as it moves through the landscape, through space and through time, you know, over the course of seasons. And these are really powerful tools. They can provide a ton of information. But in the past, they've kind of been handicapped by the fact that they're really scattered throughout this rugged mountain landscape.
And we you know, the only way for scientists to interact with them is to, you know, drive or hike or, you know, snowshoe out to them, collect their data by hand one by one, and hopefully kind of piece that together into this overall view. But luckily that is changing. And the team that is kind of behind that are a subset of the team includes Ken Williams, Kate Robinson and Andrew Wiedlea, two of whom are here today. So with that, I'll pass it off to Ken Williams, who you met earlier, to hear about what he does in this big project. Super. Thanks, Jeremy.
So again, I'm Ken Williams. I'm a geologist by training. I'm actually talking to you tonight from Colorado, where I oversee all of the field research activities at the watershed that Jeremy's been describing. And so I think it's important, actually, to highlight one really distinct aspect of what we're doing. At the end of the day, if you want to understand how, when and where snowfall changes and climate change impacts snowfall impact water flows, you have to pick an experimental catchment. And so we've settled on the East River in the area of central Colorado near the town of Crested Butte, because it's actually very representative of other watersheds within the state of California, throughout much of the Rocky Mountains.
And it's emblematic of kind of the future changes that are likely to impact snow accumulation and water exports. So we're not really overemphasizing the importance of our specific steady watershed other than to really emphasize that it's a representative watershed. It's a place where we can really make investments from data gathering, numerical modeling that Jeremy touched on, and now this really important testbed demonstration of how to actually get the data from where the measurements are made to where we're located so we can actually begin to understand its implications.
And so where we're working is really meant to be emblematic of other watersheds like this throughout the Colorado Rockies and other mountainous systems, as I say, the Sierra Nevada. Very cool. And can you tell us a little more about what the I guess, really the you know, the day to day or the moment to moment activit of collecting data from these stations and from around the East River has been. Yeah, for sure. Thanks Jeremy.
So there's a couple of things. One, you're looking at a dilapidated version of my Toyota Land Cruiser on the left, battling outdoor condition while trying to reach important field study sites. It can be challenging at the best of times. At the worst of times, it can be absolutely impossible to collect data. On the right is kind of where we've been. That's been the traditional model.
And that's a system where in we have a variety of measurements. For instance, we want to understand kind of what the saturation state of soils beneath a very dense conifer forest look like as a function of time Do they get wetter during certain times of the year? Are they drier during certain times of the year? How did they respond to big events like a giant rainstorm, for instance? Will we collect that data autonomously? And so there's a network of sensors that are recording measurements, for instance, of of soil water saturation. But that data isn't necessarily available to us in real time.
So individuals such as myself and our network of extended field based technicians have to periodically go out and retrieve manually retrieve this data. It's fine. It's the way the world works. But unfortunately for us, we would, we'd love to get to a point where we have access to that data in real time. Amazing.
And with that excellent segway, I will get to the next member of the team, Andrew, to talk a little more about what he does. Yeah, so a little background. So I'm with something called ESNet - we're the Energy Science Network. And we are the high speed fiber optic network. And there's a map of it
behind me that you can perhaps see. We connect all of the DOE laboratories and user facilities around the complex, and then we connect to sort of colleagues in research networks all over the world. So we move. Our job really is to move data collected as part of experiments, things like high energy physics, cosmology, all kinds of things.
And traditionally, we've been very focused on fixed sigh kinds of things where you have data being produced at a large scientific facility that has to be moved. But increasingly, as we all know, things like wireless and Internet of Things are letting field scientists like Ken deploy all kinds of instruments out into the world to measure what's happening, climate change and earth and environmental stuff in ways that we never could do before. So this is very much a new business for us to get into, to figure out how do we pull that fiber optic network we have out to places where we can't use fiber, but we have to use wireless instead to move the data back to the US national laboratories in supercomputer centers that we use to do the numerical modeling and simulation that Ken spoke of. So very much this is an opportunity for us to collaborate. So Ken has and the folks working sort of at the watershed field site have sensors deployed sort of throughout the environment, collecting all kinds of different things weather conditions, hydrology, atmospheric impacts, solar radiation, all kinds of things.
And so really, it's a lesson in trying to figure out, okay, how do we then build the capabilities to make it possible so that he doesn't have to rely on his folks who work with him don't have to rely on Land Rovers and hiking to pick up SD cards from sensors out in the field. But we can instead knit these together into a real time network. So that's what we're trying to do. And this is a really exciting time. from a technical standpoint, from a technology standpoint, to be able to do this because there's new options that let us communicate out into the world in ways that we were never able to before.
So I'll pass it off to Ken to talk a little more about how that actually works here in the field. So this is what one of these sensor stations looks like, kind of in a diagram view. Can you tell us a little more about what we're looking at here? Absolutely, Jeremy. I know there's sort of iterative scrolling that you can do while I'm talking, but at the end of the day, what we're interested in is what causes a snowflake to fall What happens when that snowflake reaches the land surface or gets stuck in trees and limbs of trees before it melts? And so what happens after that snowflake melts? And so it's got a variety of pathways it can take less frequently, can make its way immediately to your local stream or a river But more often, the fate of that snowflake actually is a downward progression. It melts on the land surface, begins its journey dow into the soil environment.
Where you're looking at here on the right hand side is a 3D reconstruction of a cube with that nice green land surface of lush vegetation, a nice big Aspen tree, in this case growing on the left hand side. And that dark brown is what you would typically think of the soil when you're out in your backyar and the soil there. Well, there's more to sort of the story of water beneath our feet than just that brown soil layer We may actually move into other compartments, if you will, sort of like a kind of a bank account with different accounts within that system that we need to take account of the amount of water that fills each of those accounts. And so we install infrastructure and equipment that allows us to measure how the water from that melting snowflake makes its way down through the soil and the underlying bedrock.
And so there's a really important relationship between overlying soil and underlying bedrock, specifically what we call the weathering zone. And so our work is really designed to understand what happens when that snowflake melts and moves downward through the soil, the weathering zone, and ultimately the bedrock. Well, what happens at that point the groundwater associated with this below ground processe as a variety of trajectories, a large fraction of it ultimately makes its way back down to feed streams and river flows.
But a very, very significant portion of it is actually utilized by vegetation such as that nice green layer of grass, but really importantly, these trees. And so we actually spend a lot of time instrumenting and making measurements of when and where trees are utilizing that below ground resource of water. And so we've established a network of monitoring sites throughout this very representative watershed that allow us to track the fate of the meltwater from that snowflake as it moves downward, and then in many cases moves back upward. When trees start using that water. As trees
use that water, that water now moves back up is respired by trees is a part of photosynthesis and has been is again turned back into water vapor for the atmosphere. And so trying to understand how different parts of the landscape, areas covered by grasses and aspen trees that you see here, compare to other areas of the watershed that are dominated by evergreens snow conifers, pine, spruce, fir trees, allow us to understan differences across the landscape, particularly in terms of the predominant vegetation type grasses, aspen trees, conifers, utilize water and either allow or prevent that melting snowflake to ultimately find their way back down to feed stream flow to the bigger Colorado River system that Jeremy presented earlier. And so different trees and we're looking at trees right now and respect the conifers exert different influences on how that snowflake makes its way to the land surface.
We're all familiar with the concept of an evergreen. They're evergreen because they never lose their needles for the most part And so a lack of losing needles actually serves as a great interceptor for snowflakes falling down through the winter and spring that keeps those snowflakes from reaching the land surface, melting and driving that flow of water through the soil, weathering zone and bedrock. In contrast, areas of grasses and aspen tree exert a very different control in their ability to get snowflakes to land surface.
And so having instrumentation across the diversity of landscape types in terms of conifer, deciduous forests and grasses is really critical for understanding the fate of water tied to that snowflake. Cool. So you basically have these weather stations tied to deep wells that are monitoring what water is doing underground tied to plant trees, sensors that are actually monitoring how that water is moving up into vegetation. And altogether, that creates what you call a bedrock to canopy view of how water is acting and how specifically how it's acting in each of these kind of unique biomes you've identified, whether that's deciduous forests like aspens, whether it's conifers like pine trees or mountain meadows or alpine, which is, you know, above the trees all together. So tell me a little bit more about what the importance of groundwater is in all of this.
I feel like that's kind of the invisible, you know, an invisible factor. It's not something most people think about that much. Yeah. No, absolutely.
It's literally the water beneath our feet. And it's actually a really, really important component of flow in rivers that we're familiar with. Walking around in our backyards, walking around in the Colorado River Basin, you see screen flow and it, of course, varies over the course of the year.
You know, during the period after snowpack begins to melt, we see really big increases in stream flow. As that snowpack melts out and we move into the summer and fall season, you still see flow and streams the vast bulk of the flow in those streams actually supported by groundwater. And so if you don't have an understanding of how groundwate both accumulates, tied to recharge from snowmelt and then discharges to stream flow, you're missing more than 50% of the water story in these mountain systems. And so having measurements that allow us to track variations in groundwater from year to year, season to season is critically important to understanding how these streams will respond to climate change in the future. Interesting.
Okay, cool. That's that's such a it's such an honor being a part of it all But it makes sense that that is ultimately a really big part of the picture here. So to get a picture of the entire watershed, you obviously have this really scattered network of sensor systems. There's obviously, as you mentioned, with your battered Land Cruiser there's a lot of challenge inherent to constantly going out, traversing these mountain landscapes and accessing all of these independent sensor stations to, you know, get them with a thumbdrive and download that data.
What is the kind of what would make that process better? Well, access is a huge issue, while the snowpack is the central story in terms of how much water leaves the watershed. It presents really big, you know, sort of roadblock for our ability to rapidly download the data from this big diversity of sites. Here's my site in a conifer forest. Here's my site in a grassland.
Here's my site in Aspen forest snow in wintertime conditions make it really hard to get access to that data. And so what we really are interested in coming up with approaches that allow us to have insight in what's happening at a given location, at a given point in time, in real time, in the same way I can pick up my phone right no and say, Wow, I just got an email message. I would love to have an observational network that alerts me to say, Wow, something unique and interesting has just happened at your site so that I can then respond in a very rapid and efficient fashion. Very cool. Okay.
Well, that brings us to what Andrew and folks at ESNet are doing to kind of solve this problem of access. I'll pass it off to Andrew. Yeah, absolutely. Really, our role in this process is to try and buil Ken that near real time network that he and other scientists need. And that's enormously challenging in this kind of area because, you know, you're dealing with a space where communications may be very limited. There may not be cell service, there may not be any kind of real good radio coverage at all. And so what you have to do is figure out how you're going to connect these network of sensors that may be scattered out through the landscape.
And some may be underground, some may be attached to trees, some may be a distance from from each other, and try and create a capability for those to then in near real time , communicate and move their data back to the database or the model or wherever, whatever use is being made of that information. So we've been approaching this problem sort of a couple of different ways, and this is very much sort of developing technology with a couple of different pieces. And again, there's no right one answer to it, because different sensors had different capabilities.
They have different availability of power or they have different data rates, different kinds of information that pass back and forth. So what we've been working to do is deploy something we're calling a senso distribution station, which is a solar powered unit that supports a variety of different sort of local wireless standards. So Wi-Fi like you might have in your home. LoRa, which is another standard for long range communications to mesh network capability.
But there's other types of standards we'll be deploying based on what each sensor can use and how it can communicate based on what its constraints are and line of sight and stuff like that. So you have this local sensor station, and then that local sensor distribution station is connected via a private 4G or 5G network, basically like a private cellular service that we're deploying in the area to be able to sort of paint a wider area of the landscape. And this is something that's very new for us. at ESNet.
We haven't operated sort of wireless equipment in this way before, but that lets us basically operate very much like you do with your your handset at home, except we're communicating with it with a fixed solar power station. Then the next part of the problem is, well, we don't have fiber optic at the location where the cellular network is operated from. So, you know, you think about your local cell tower when your phone communicates with that cell tower, it's actually going onto then a fiber optic network that's connected to that cell tower. Now, the problem we have in this place is there is no fiber optic. So there's no high speed connection to get data back from the top of the mountain where it connects to the cell tower back to the lab. So in order to do that, we've had to make use of a new capability called Starlink, which is a low-Earth orbit satellite constellation.
You may have heard about that. It's coming online now, and many people are using it in all kinds of different settings But it's very exciting technology. And so we're using this low earth orbit satellite constellation to then backhaul, as we call it, moving data back the data from the cellular network that we have operating in this remote area back to our fiber optic network. And it connects in Seattle and then move that data from Seattle to Lawrence Berkeley National Lab or wherever it needs to go.
So that's the basic architecture There's a couple of pieces that will all have to come together to work to make this backhaul and two way real time comms happen. That's awesome. Can you walk us through how this actually looks out in the field? Sure. Absolutely. So right now we're at a meadow called Snodgrass. And there is actually if you look in the foreground right here underneath the trees, you see a couple of different sensor stations. And those are collecting a variety of different environmental and hydrological and other kinds of data that scientists need.
Off in the distance, right here, actually, you'll see actually right behind me. That's great. This is the solar powered data collection station. So this is operating in that area and it's providing WiFi and LoRa and other services to sensors that are in the immediate area, sort of within line of sight. That's basically the router.
That's the router. That's the first step in the data journey. And then if you go back real quick, Jeremy, back to photos real quick.
Yeah, perfect. So what you can see in this thing off in the distance here, I don't know. You can't see my pointer, but there's a little white spot there. Go right there. That's actually where our cell tower is located So the sensors there is actually connected on our private cellular network And it's sending the data to this, which is the other side of that white dot on that hill. And this is an atmospheric radar monitoring station run by a different program, but it's a metereological radar station. And then on the other side of this round dish, which is the radar actually go back, if you will, right on the other side.
You can't see it's hidden behind the radar here. There's actually a cellular antenna that's on the other side of that of that catwalk there underneath the radar dish, which is the round dome. Anyway, that's collecting the data from that area called Snodgrass that I spoke of. And that data is coming down and coming into the trailer. And then right in the foreground, right here is the Starlink antenna, and that Starlink antenna is passing the data to a satellite in orbit that is then sending the data to Seattle, where it enters our fiber optic network. And you can see behind me on the side, I can never I can never do the point that Seattle up there and that's where where things come to ground.
Oh, there you go. Perfect. So there's kind of a complicated path for like all those hops to happen for data from that sensor to go from the sensor to the wireless collection station on Snodgrass to the cell tower to the Starlink to Seattle, and then into yes, that's fiber optic network where it comes to Lawrence Berkeley National Lab, which is the yellow dot on the left side of that map right there. So that's the path and how it works.
And we're very excited to sort of make these things all work together and get these technologies, many of which are very new, working together. That's amazing. So after all of these steps of, you know, you basically attach a go out to all these sensor stations scattered throughout the field and you create kind of a central router for each of them nearby so they can all be wirelessly connected to that router then beams all of that information to like a central radio ad with a satellite upload, goes up to space satellite fleet, sends it to Seattle. And Seattle finally, where you have this actual plug in to the fiber optic network, which is kind of the superhighway of ESNet. And at that point, finally, any scientist anywhere in the world can can be like plugging right into this.
Exactly. Exactly. And that's the vision. That's what we're working towards. And the idea here is that we want to be able to provide Ken and other scientists with near real time data. But we also want to have them have the ability to, say, control their sensor systems without having to drive that into the field. And particularly when it's, you know, when there's snow on the ground, that can be particularly tricky Oh, cool. So it's not just like a one way, you're not just reading, you can also kind of send back instructions of like, you know, collect, collect information or change the rate of data sampling.
Exactly. That's what we're working toward. And then sometime in the future, it may be possible to fly a UAV, for example, just like the one you flew Jeremy to, to make some of these photos. And actually that we can use that to move sensors around, for example, on the hills without having to have people do it by hand. That's awesome. So why? Can you can you tell me a little more about, like, what is, I guess, first of all, like, what do national labs exist to do? And, you know, how does this work fit within that kind of broader goal? Yeah. No.
So the US National Lab System is really wonderful it's one of the big backbones behind sort of how our nation has become prosperous and many of the big scientific challenges that we've had to face. So, you know, the national labs exists to basically help the country manage hard problems. So hard problems like the climate is changing, hard problems like we need new sources of energy, you know, hard problems like, defense problems that we have. So, you know, we cover all kinds of different activities and in particular at Lawrence Berkeley National Lab, everything we do relates to sort of basic science. You know, we're really it's the lab is set up to help teams of scientists understand the fundamental properties of the universe and our planet and to help them understand, like, where we're going and how we can how we can end up in a better place than than we might if we weren't managing the problem.
Really cool. And I'll take a moment to pause and just say, anyone in the audience, you're more than welcome to submit questions about anything that is unclear, anything you're wondering, or want to hear from Ken or Andrew In the meantime, I'll actually Ken, I'll bring you back. I'm curious to hear, you know, within this national lab system, how does fieldwork work? Like, who do you collaborate with, what you know, what are the parties that kind of go into the work that you do out there? Yeah, thanks so much. So, I mean, one of the big aspects of doing national lab science is the science is what we call team based science.
And so to really understand how a watershed works in its ability to deliver water now and the future requires a team of scientists, really a team of teams. You need people that are specialists in vegetation, right. How are these plants utilizing water? You need experts in hydrologic modeling. How do numeric models describe how water flows? You need atmospheric sciences scientists that really understand how, when and where snow is and precipitation are falling.
And so what we do is we really serve as what I call a community watershed to actually host the activities of not just national lab scientists, but other scientists with actual expertise that we need. Folks from the U.S. Geological Survey, folks that are specialists in atmospheric measurements, folks that might be specialists in microbiology, that you would think: Why is that important? Well, microbiota really control the the behavior of vegetation to a large degree.
And so to get to Jeremy's question, it really when we create a research experimental watershed session, what we have in central Colorado, it necessitates a team of teams. Those come from both the national lab system, as well as our partners at the university and other federal agencies. Very cool.
What, Andrew or, you know, either of you. Could you tell me a little more, I think you mentioned that this is really the first time that ESNet is branching into this realm of like, you know, how can we connect kind of small, scattered things in the field to this big set of infrastructure? What do you imagine is kind of the future of this? Like, what are some of the other applications you can see? Yeah, absolutely. And actually, that kind of relates also to one of the questions that just came up in the chat.
Someone asks like, how difficult would this be to do it in another area? And the answer is, you know, this is very much, you know, we're in the process of learning. So we're in the process of deploying and understanding how we want to build. This is really a long term capability that's connected to ESNet and the science that we support. So, you know, in terms of, you know, the deployment, you know, really, that depends on the specifics of the geography, the terrain, the vegetation, the number of sensors, how things are working there. It's hard to answer that directly.
Certainly, it's very easy to set up Starlink and work sort of anywhere in the continental U.S. and abroad. But the terminals themselves are fairly expensive and fairly power hungry, which is why you don't use them to directly support the sensors in the field. So then you need to figure out, okay, so how do you want to manage the coverage in the local area? And actually, in many ways the trickiest part of this whole problem is the last 500 feet, more so than the other 1500 miles, because it's really you know, every sensor is different. Every sensor has its own data collector.
They have their own protocols. They're built there for their own purposes. Very often they're very constrained in terms of power that they have available or what they're able to do. And so really, one of the big lessons that we're learning is, is not so much that hard problem of the first 5000 miles is that last 500 feet.
And how do we deploy technologies that are suitable to operate, you know, unattended in rugged, remote, rural areas with very little power use and very little attention? And that's really the hard part. But I think I think we are trying to work our way towards something we're calling like a wireless data transfer node, which is a standard for how this might work. And I think as that comes together, then we would be looking to expand, you know, how we can offer this to other people. But it is now we're sort of entirely driven by scientific need. We exist as a support function for the scientists who have to deploy things. Awesome.
That is yeah, that's so cool. And it just seems like a, you know, the kind of dawn of a new era of like what's possible in terms of this field work that has typically been so constrained by the existence of existing infrastructure. Another question that was rolled through in the chat is about the implications of this for management, and I think that it will actually be best answered if we go back to Ken to talk about why it matters, like what you know, what are the actual implications of this beyond just convenience and, you know, the ability to to get out to these field sensors more often. Yeah. Great.
I was busy typing a reply to that Q&A in real time. So you caught me a little off guard and so absolutely. So traditionally the approach has been and you'r probably familiar with this is we have a network of U.S. Geological Survey funded stream discharge monitoring locations.
And so we can look in the course of a day or a week or the past month or year or in some cases hundreds of years What are the streamflow behaviors over the course of the year? How much water is coming out of the system? Because at the end of the day, that's what really matters for downstream users. Jeremy touched on when we introduced the the web-based Story Map, you know, the importance of this color of water to locations as far downstream as the Imperial Valley for growing much of our wintertime agricultural crops for lettuces and for other vegetables. And so understanding over the course of the year how much water is actually goin to make its way downstream for users to use is critical. And so the USGS has been great in installing discharge monitoring stations. So how much water is flowing past a given point on this river at any point in time? That's great.
What we've tried to describe and what we're really intereste in is all of the, if you will, upstream activitie from plants, from the sun, from other factor that impact how much water makes its way in the system. We need to also make those measurements as well, because it's not just streamflow that's critical. It's understanding how the entire system behaves in an interconnected fashion. And so the measurements that we're making are important both in terms of immediate, actionable intelligence, how much water is flowing, how low or how high groundwater is. But really, it's the data that we need to feed into these predictive models that say, Aha, we got this much snowpack this year.
Here's how much you Imperial Valley farmer can expect to have this year. And so it's this compilation and assimilation of very, very different data tax, not just stream discharge that are critical for the long term decision making to understand just how much water we all have to make do with going into the future. Really cool. And I mean, how how does this new kind of era of like having connected networked together sensor systems and therefore like a real time constantly updating data stream, how does that change the paradigm of this, you know, this mandate to be able to to predict and help manage downstream flows? Where, when and how things happen.
And so if you don't have an understanding of where the most important parts of a given watershed, you know, if you don't understand where the most important parts of the watershed are in terms of regulating the ultimate discharge to streams and, you know, eventual delivery, say, down to our Imperial Valley farmers then you're missing a really important part of the story. And so, again, going back to just measuring stream discharge, that's great. But if there are certain parts of a watershed or certain parts of the landscape that are that have an outsized importance in terms of providing that stream flow, if you don't have the active measurements to understand how they're behaving, you're missing a critical and central part of the story. And so what we're trying to do is, again, deploy measurements in monitoring across all the different components of the landscape with this critical sort of additive component that allows us to make these measurements and understand how they're changing in real time.
How do they vary in April? How do they vary in May? How do they vary in September? That's the big sort of nut we're trying to crack because that's at the heart of understanding which parts of our watershed are most vulnerable to climate change and how those ultimately impact the delivery of water, not just to our local communities to those far downstream. Really cool. Andrew, this might be more of a question for you, but you know, I imagine the more sensors you have and also the more kind of variables that are monitoring, it seems like you you very quickly start to have this kind of pour of data, this massive body of information. How does you know really the net this kind of growing, you know, age of having a huge amount of information and increasingly computers that can process it. How is that plug in to all of this question of just like infrastructure? Yeah, I know. That's a great question.
It really is the primary motivator for a lot of what we're doing here. You know, just on the sort of optical traffic side, our traffic grows by a factor o is growing about 60% a year. So it's, you know, sort of exponential growth. And that's also happening in the field science community. What's also happening is, as you imagine, start to imagine a world where sensors are deployed sort of much more widely, collecting much more data. You're also going to start to see changes in how sensors are used.
And that's getting at the sort of Ken's point about, you know, the improved ability to manage things. Not only can you measure things in real time but you can also begin to respond to them in much faster than you ever could before. So, you know, in terms of in terms of what that means for the network, it means, you know, we're running as fast as we can just to keep up. Right.
Our job, ESNet's job is to free scientists from geographic constraints and make it possible for folks to really focus on their science and what they need to do. And so this makes it sort of essential for us to explore this wireless space, to understand the kinds of capabilities that are coming online, and then to figure out what the service model is going to be for us, for scientists in terms of deploying these sets of capabilities or leveraging commercial systems like Starlink, where we can to help make the job easier. Cool. Ken? Yeah. I just wanted to add one thing, you know.
Even ten years ago, I had no predictive power over what type of sensors were now suddenly going to be available to make measurements that might be of interest. And so while we haven't highlighted here one of the other histories that this particular watershed is a long legacy of mining and metal impacted waterways. And so can we come up and do we have access to sensor that have the ability to potentially measure sudden changes, for instance, in heavy metal contamination of our waterways? If that's the case, that's really important information to get out in front of in real time.
Why would you be interested in saying, Yes, our sensor measured a sudden discharge of some, you know, really deleterious metal due to a river environmen and discovered that only two months later. Why not have the ability and work towards solutions that allow us to say, oh my goodness, we've just discovered a really important environmental event. I'm giving you an example of a metal release. For instance, we saw this in southern Colorado tied to the to the Gold King Mines Bill. It's really important to think about sensor technologies that don't even exist yet, which may provide immediate information about how the environment is responding that allow us then as scientists and eventually, you know, water users, downstream users and water regulators to act upon that information in real time.
That's something we haven't had really access to in a meaningful way across these really remote locations until the advent of this project. That's very interesting. It strikes me, too, that that is related to like a climate change future where, you know, you're having increasingly intense and unprecedented events like, I don't know, a really, really unseasonably warm day in March, you might have this massive influx of meltwater that, you know, suddenly downstream people need to react to, but they can't do that if they're first, you know, their first inkling of it as the river swells it massively. That's a great example. If you're at the point of looking out your back window and seeing the rising floodwaters, that's too late.
If you have the ability to actually tie into a real tim network of information that allows alerts to be had, for instance, to use Jeremy's example, let's say we're in lat March and it's a very warm day and we get a very heavy precipitation event. But it's not snow. It's rain. That snow drives very, very, very rapid melt in a way that sends a lot of water to streams, that has the potential from a flooding perspective to impact downstream communities. And so the ability to actually ingest or take in as much information as we can, even in locations that are so remote, they've had no access to any other form of telecommunications. That's where the added value comes, I think, in terms of the relationship of our science to the broader public.
That's really cool. Well, I think that's as good a segway as any to talk about what, you know, the future of at least river science in, you know, the Rocky Mountains might look like and what some of the other areas you're looking to branch out into are. Yeah.
And you and I talked a bit about Union Park Sure. Yeah, absolutely. So, you know, as we begin to think about, you know, parts of our, you know, global network of watersheds that are being heavily impacted by climate change, we can actually look for what we call some central sites. So sites that, you know, locations in a watershed or in a mountainous environment that are very likely to be impacted by climate disturbance Maybe that's massive wildfire. We've seen a ton of that in California, obviously. We've seen a ton of that several years ago in Colorado.
But there are other drivers that are impacting our forests. Right. As temperatures rise, you see increased impacts of mortality for trees driven by insects. And so can we begin to think about a future world where we're out in front of managing our forest to try and minimize these giant deleterious impacts Can we actually proactively manage our forests in a way that improves their resistance and resilience to things like wildfire and insect mortality? If we can, can we begin to look at the consequences of actively managing our forests on water resources? I think we can, but the ability to actually relate those measurements, right, or those activities managing of forests selectively, logging to improve forest health, running prescribed burns to link then to that activity to water export. How much water leaves the system that's going to require active measurements, that's going to require this expanding network of existing and to come future sensors that understand the relationship.
That's where we want to be. Those activities are occurring in places where we have no chance anytime in the near future to tie in to existing cellular networks. And so the work that we described here today, we think, is the next step in bringing measurements to active land management to improve forest health and water resources. That's where we're going and that's where I think this work really represents a foundational first step. That's so cool. Amazing.
Well, that is an awesome overview of just kind of what this work is and what it might mean for, you know, really everyone who depends on the Colorado River and the future of understanding these mountain watershed systems. I want to take a moment to just acknowledge what I thin has already been touched on a couple of times explicitly which is just that like this this type of collaboration between, say, like a geoscientist who works predominantly in the field and a network engineer who works, you know, abstractly on this. Like, you know, a worldwide system of infrastructure is not a no brainer. It's not a given, especially, you know, in academia or private industry as it exists. So I think it's a really cool thing about the national lab system.
And specifically Berkeley Lab has this really cool legacy of team science and the idea that you can get multidisciplinary groups of people working together all under the same roof. So, you know, just a kind of a shout out to Berkeley Lab. And I want to bring in Faith, Director of the K-12 programs to talk a little more about how people can get involved with working at Berkeley Lab and how that kind of starts from a really young age. Sure. Thanks, Jeremy, and thanks, Ken and Andrew for your great overview of the work and research and making it accessible to everybody tonight For Berkeley Lab, we are very enthusiastic about developing the future of STEM and especially giving students an opportunity to see themselve in STEM workforces, STEM workplaces. So our K-12 team actually has a high school internship program, as well as some camps.
Starting with we're opening up our applicatio for our bilingual STEM camp in April on December 5th. We'll be openin young women and those that are non-binary in January. And our internship and pre-training internship program in February.
So we're going to be busy over the next couple of months recruiting students and making sure that they get the opportunity to see themselves for as little as three days, five days, and a whole six weeks here at the laboratory. And we know that those students can go on to the DOE funded undergraduate, graduate and community college programs that are supported and hosted by our Workforce Development and Education Office here that Alisa is putting a link to in the chat box. So we're both working together to make sure that students get an opportunity at a very early age. And we are so grateful for all the scientists, researchers and staff here at Berkeley Lab who support all of the students through their volunteer efforts in service across the laboratory with the 4000 employees and 1700 scientists and researchers.
So that's the way that people can get interested and get started and start off their career at one of the DOE National Laboratories. So thanks for allowing us to talk about that and I'll pass it back to you, Jeremy. Yeah, and I, for one, can speak firsthand to the, you know, the value and the power of these internship programs, because I myself came to the la as an undergraduate intern actually working with Ken Williams, of all people. So it's a really cool resource that exists. And we hope that this Story Map and kind of communication tools that help encapsulate some of the work that's being done at Berkeley Lab can be a tool for folks in that, you know, that goal of educating about what kind of research is being done in institutions like this and how it impacts people like the 40 million folks, ourselves included, who depend on the Colorado. With that, we're just about out of time.
There is actually one more question in the chat that I thought was really interesting we didn't get to. Ken, can the sensors be used to measure soil dryness And how does that have an effect on wildfire? I'm sorry. Wildfire burn and soil sterilization. Yeah, it was a great question.
And so, yes, that's one of the sensor technologies that we have, you know, sort of well and firmly developed over the past 15, 20, 30 years. That's the ability to monitor variations in moisture content in soils. And so as soils get dry, vegetation become stressed, vegetation when stressed moves toward a model of let's shut everything down. When vegetation moves to let's shut everything down model, it becomes much more susceptible to heavy burn and severe burn. And so very wet vegetation, any of us that are campers or, you know, even of our fireplaces at home trying to light a wet stump on fire or wet twigs on fire is really hard. Trying to light dry, brittle, you know, woody biomass on fire is really easy.
And so the ability to monitor variations in soil moisture content or dryness at multiple locations, not just one or two, like everything we're talking about today, is designed to expand our network of monitoring locations at skills that matter, to capture all that variability in a very complicated watershed. You know, that's where we're trying to go. And so the, you know, with respect to soil moisture and soil dryness, capturing where and when soils are abnormally dry allows us to think about fire potential in a way that's very different than not having that information. And so worrying about parts of the watershed that might be on fire or soon to be on fire tied to a fire event that are already pretty wet. Maybe that allows you to marshal your resources in firefighting to areas where your sensor network says, Oh my goodness, these soils pre fire are extremely dry, extremely arid, and more likely to lead to catastrophic wildfire. Great question.
Cool. Awesome. Well, with that, we're at the end of our hour and I'll let everyone go. And I'll just highlight the final aspect of the Story Map, which is these resources at the end here are designed to kind of be educational resources for folks as well as links to all of the websites of the organizations mentioned here like ESNet, like EESA and Berkeley Lab. So thank you all so much for attending and thank you, Andrew and Ken, for being here and being involved in this work It's been awesome to learn about it. Well, thank you, Jeremy. This is awesome.
Really fun to do. And thanks for the great Story Map. It's really, really cool to see. Of course, I would say this is you know, I'll let me just thank Jeremy as well and I've worked with Jeremy for a long time, will continue to be working with Jeremy for a long time.
These Story Maps are the future, these Story Maps are absolutely the essential linchpin between communicating what's often very complicated and impenetrable science and engineering to the audience that matters. The individuals that actually rely on these, in this case, water resources. And so I thank you, Jeremy, for really spending the time to try and present complicated ideas, complicated measurements in a fashion that we can all understand. Thanks again. It's an absolute pleasure. And Alisa just put the link to the Story Map in the chat.
We'll definitely be sharing it out through our channels in the coming weeks, but I hope that you all can take the time to go check it out or share it with anyone who might be interested. All right. With that, I think we'll call it a night. Thank you all so much for coming.
Thank you all. Thanks all.
2022-12-10 04:39