Advancements in ESI-TOF MS Technologies for Greater Understanding of Biologics
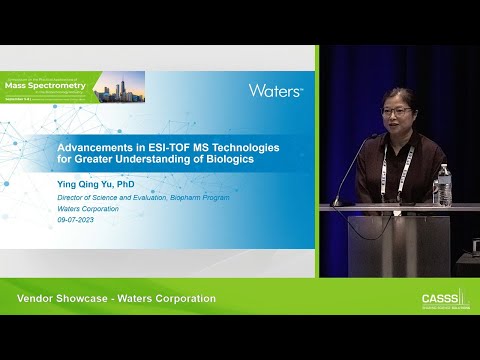
Good afternoon. My name is Ying Qing Yu, I'm from Waters Corporation. I'm a long time PAMS conference attendee, and this is my first conference since the pandemic. So it was very nice to come back and see all the familiar faces and new faces. And I would like to thank Chris for gave Waters the opportunity to present at the launch seminar, and I promise my presentation is light and easy to digest, just like your lunch.
So my talk title is pretty generic. It's pretty broad. But the focus of my presentation is on the new time of flight instrument that's recently launched from Waters, and I want to talk about its potential usage for biologic sample. And I actually was one of the few people attended first CASSS MassSpec conference. Yes. And I have a little trivia question for everyone, because I tend to remember years by what's the best box office movie.
Do you know-- anyone know? Don't, don't look at your phone. What's the number one movie 20 years ago in 2003? Take a guess. A hint, it's an animated movie. Who said Finding Nemo? Yes, you're right. It was Finding Nemo.
I went to a movie theater with my husband, and I was so blown away by this movie, it just so beautifully made. I still remember all the detail. So you know what's equally beautiful that year? Is CASSS MassSpec. At the time there were many talks at the first CASSS MassSpec conference. Talk about time of flight, instrument, and why is it superior for a large molecule characterization.
And I don't really have this slide from back then, because I just searched the CASSS website. I couldn't find anything. So I just use a figure from my application note to illustrate why time of flight instrument at the time was so superior, is that you can get a very complicated raw mass spectrum, and then do a maximum charge deconvolution, and you get net zero charged molecular weight masses. And then that tells you what type of heterogeneity you have in your monoclonal antibody. Mostly you can see very clearly what are the top glycoforms, and also sometimes you can see the c-terminus lysine conjugate. Because they can all be resolved by like say 5 to 10,000 mass resolution time of flight instrument.
So that's why it's so exciting. And then at the time I just started working at Waters, and my very first instrument was LCT Premier. And because many of the talk at the first CASSS MassSpec use the LCT instrument. LCT stands for liquid chromatography time of flight. OK.
And here's my-- I want to talk just a little bit about the LCT Premiere instrument. Because it's a very well liked time of flight instrument at many companies. I think maybe some of them companies still have them.
I hope. Why? What makes LCT Premiere so nice for doing monoclonal antibody or large protein analysis? Because at the time it has several advanced technology being built into the LCT Premiere. For example, the ESI Zspray, step wave technology, and the better vacuum. All these are incorporating enhanced sensitivity for large ions.
And also it has v and w mode. So the v mode at the time is 5,000, and w mode is one 10,000. So it's enough to get average mass measure for large proteins, such as monoclonal antibody. And also this instrument has positive and negative ion mode switch, so that allows you to analyze diverse type of compounds and also enhance your dynamic range. And since then there are many more advancements that have been built into our time of flight instrumentation with ESI source.
For example, the ion mobility that's built in-- the traveling wave ion mobility built into the instrument allows you to separate compound further in the gas phase based on their charge, shape, and the size. And then also we had compact time of flight instrument that's benchtop system, that's very robust and it can do a variety of analysis. And also in the commercialization of UPLC in early 2000, it can-- the ultra performance liquid chromatography enables to people to do more sensitive separations, that you can pack more peaks in the chromatographic run. So it makes the whole ESI TOF-MS technology much more attractive. And then, oops, sorry.
Go back. And then we also had an HDX system. HDX system is very useful for high order structure analysis.
That when you combine HDX, the robotic system with sample manager, the LC stacks, with instrument like synapse system, that you can really do fast chromatography to prevent the hydrogen deuterium back exchange, and then you can also utilize the ion mobility separation because you pack so many peptides into five minutes to run the ion mobility, separation to help you differentiate those peptides. And then also later we had a very small footprint TOF instrument called RDA. And the whole along with the LC stack in the front, we call BioAccord system. The BioAccord system even though it's very small, it's very versatile. It can do intact protein peptide mapping, release glycan lipid nanoparticle analysis, and oligonucleotide analysis all in one system.
And what makes this instrument more attractive is that it's controlled by compliance ready software. So it has the potential to be implemented into a QC environment. And then recently we also launched select series. Our top end high resolution, high performance mass spec. And one of them is called Cyclic IMS.
I think last year we had a presentation at PAMS to talk about what type of application you can do for biopharm using Cyclic IMS. And then this one here is most recent launched called multi reflection time of flight instrument. And that's the instrument I will be talking about today. Select series MRT, the next generation of Q-Tof.
This is just to give you an overall performance of what the MRT instrument can do. It is our next gen, Waters next generation Q-Tof instrument. And it can routinely give less than 1 ppm mass accuracy on average about 500 ppb mass accuracy, which is pretty impressive. And in MRT mode, you can get 200,000 mass resolution at full width half maximum. And it's irrespective of your acquisition rate.
So your mass resolution does not decrease if you're doing 10 hertz acquisition, 5 hertz or 2 hertz or 1 hertz. It stays the same. And also, you can tune the mass resolution to higher, at 300,000, using a resolution enhancement called REM. I'll talk a little bit about that, too.
And also, this instrument gives a reduced chemical background. And then it gives you very compact data file size. So you have reduced file size, compared to our other Q-Tof instrument. At the front end, it has MALDI and DESI. That's desorption ESI and also, just electrospray ionization source.
And as far as acquisition mode, you have MS, MS/MS, and DIA, and DDA mode. Now, I want to point out, when this instrument was initially launched, it was meant for doing mass spec imaging. Because mass spec imaging requires ultra high mass resolution, so that you can differentiate hundreds of thousands of small molecules that have a variety of a chemical formula, all packed together into a very small area. So you need high spatial resolution to accurately identify those molecules. So maybe just give everybody-- I still remember my grad school mass spec class, taught by Graham Cooks.
So here's a little equation for calculating the mass resolution for time-of-flight instrument. So the mass resolution is m divided by delta m and also, equal to t divided by 2 delta t. And do you know what t is? t is the flight path. The delta t is the energy spread in the ion pocket. So the way to improve or increase the mass resolution in time-of-flight instrument is you can increase the flight paths.
Now, originally, for the Linnean TOF, you have your ions being pushed and to a detector in one direction. Now, you can increase that one flight path to as long as you want. But that's not practical. You can't build an instrument like that. Then later on, we have the V mode. So V mode has built in those grilled-- grid reflection to deflect ions and make it V-shaped.
So you actually double the flight path, and therefore, you increase the mass resolution. And later on, we have the W mode, same analogy, but you quadruple the flight, therefore, increase the mass resolution by increase the time-of-flight path. But those grid here, if you put a lot grids here, you start to lose ion transmission. Each one, each grid, will only transmit about 92% of the ions. So if you keep building the flight paths using this method, you're going to eventually lose all your ions. And what the multi-reflection technology, the engineer with a newer technology called a gridless mirror here.
So it minimized the loss of ion transmission. And also-- I don't know if it's here. Also, in the middle here, you can see those are called periodic lens, that it can improve the ion spread in Z direction. So it focused a little bit better. That's also improved transmission. And also, in the last one, last periodic lens will bounce the ions back.
So it come this way to the last plate, and it bounced back, hit the detector. So overall, the entire flight paths in the multi-reflection mass spec is about 48 meters long, which is really long. And that's why you get this super high mass resolution at 200,000. Now, remember I mentioned this ultra high. The 300,000 mass resolution is also achieved by doing a multipass multi-reflector mode.
All it means is that you do this one round. Then you do another round. So you do two rounds, and you get another-- oops, sorry. Didn't know there was an animation here. You increase another 100,000-fold mass resolution.
So those two modes depends on your application. You can just stick with the regular mode, or you can go with the REM mode. And there's actually more modes in the instrument. So for the people doing proteins, peptide analysis, you probably care this most. So for protein mass spectrometry chest, MRT instrument has-- you can use the MRT mode if you analyze less than 10,000 dalton. But there's also a improved MRT mode, called MRT protein mode.
It improves ion transmission for proteins or peptides greater than 5,000, but less than 50,000-ish. And you only suffer slight reduction in mass resolution. So you go from 200,000 to about 170,000.
I can talk about why this mode is interesting. And the last one is more of just a diamond mode, meaning like your ions get pushed and then go through one pass and hit the detector. So this diamond mode is for really improved ion transmission for super big molecules, like monoclonal antibodies size.
So anything greater than 10,000, you can also use the diamond mode. Here's just an example of why ultra high resolution is very useful. So this is a small drug called I can't even say. It's sulfadimethoxine. It's a small molecule here. And then if you run on typical typical TOF instrument, you get an isotope distribution.
And the tallest peak usually is the most abundant isotope, a combination of them, like C12, nitrogen 14, oxygen 16, and so on and so forth. And then you go through your isotope-- sorry. You go to your isotope theory. The next one, often, people just assume that C13 peak. But if you look at the data on the MRT instrument, you zoom in, you actually see three peaks-- oops-- A, B, and C. So C, I can't see it from here clearly. It maybe has C-13 isotope.
But the and may have sulfur 33 instead of sulfur 32. So you get more of a combination of different isotopes in fine details. And then same thing for this last isotope peak-- so there's a lot more than just C13 in your sample.
And that's the power of high resolution. Now, I switch gears and talk about how MRT can be used for monoclonal antibody characterization. OK. This is an example of a peptide mapping data.
What you see here is that we can just routinely get 98% sequence coverage. And if you look at the results from the readout for the top 100 peptides, from the data processing, you get delta mass error less than 500 ppb, right here. So on average is 485 ppb. And the top 1,000 product ion has a mass error of 0.5 millidalton, so it's pretty impressive. And oh, by the way, you can run a very short peptide mapping gradient.
And you can still maintain a very high sequence coverage. Here's an example of deamidation. So if your regular unmodified peptide and deamidated peptide or co-eluting, so meaning they're sitting on top of each other, if the instrument has low mass resolution, you're probably not going to see the two forms.
But in just a regular MRT mode, you can start seeing the deamidated peptides being pulled away from the non-deamidated peptide. So the blue dots is the isotope series for native peptide, and the green dots are for the deamidated peptides. You can start seeing them being split apart right here. It's almost baseline result. By the way,
this is a 2+ charge ion at 542 dalton. And here's another example. That's a triply-charged peptide. You have a blue series that's unmodified, and the red series are-- I'm sorry. Yeah.
OK, unmodified, versus deamidated form-- and you can start seeing in the green trays here, peaks are being resolved at 200,000 full-width, half maximum in MRT mode. That's very good already. And if you turn the REM, mode, that's the multi-pass, multi-reflection mode, you can start seeing they're being baseline resolved. So this is just to demonstrate the power of high mass resolution, what it can do for differentiate the co-eluting deamidated peptides. And I think, from my recollection, I can see them being separate apart for ions as big as 1,000 AMU, but doubly-charged peptide at m over z at 1,000.
All right, so maybe I'll just skip this. And here's an example on subunit analysis for mAb. And this is phabricator digest monoclonal antibody. And you have the half FC light chain.
And the FD subunit's been separated on LC. And from the raw spectrum, here's the whole charge envelope for each subunit. But if you zoom in to a particular charge state-- I'm sorry, this is the raw spectrum. You can zoom into the charge states. You can see the-- I'm sorry. This is a zoom into charge state.
So you can see the [INAUDIBLE] isotopically resolved species for all the subunits. And the molecular weight is about 20 plus kilodalton. And they're all being isotopically resolved.
And the mass accuracy is very high. So it has very, very low ppm. It's hard for me to read, so somewhere between 0.4 ppm to maybe 2 parts per million. So for the subunit analysis, you get very, very good mass resolution and the mass accuracy. Here's another example of light chain heavy changes by adding DTT and a monoclonal antibody.
Oh, this data is collected in the protein mode. The heavy chain is about 50k dalton. Both subunits, here's the charge envelope.
And if you zoom into the light change, oh, particular plus 18 charge states, you can see the isotope distribution that's being completely resolved. And after charge deconvolution, you get 0.7 ppm mass accuracy. And here's the heavy chain again. It's all isotopically resolved. OK.
And then the maximum 1D convolution gave you distinct glycoform. I didn't have mass accuracy, but here's the less than 2 ppm mass accuracy. And here's the intact mass. Remember, really, really big species, you want to use the diamond mode. And this just to show you the quality of the raw spectrum from a C4 separate monoclonal antibody.
And here's a zoom into one charge states. You can see clearly the glycoform distribution. So it's still very good performance, but it's more like a regular time-of-flight instrument here. And I want to show some examples of top down and Native mass spec.
This work was done in collaboration with Professor Joseph Loo, from UCLA. Here is a carbonic anhydrase sample. And when we perform the top down, we did the top down on the denatured carbonic anhydrase. And we tried three different ways to do top-down acquisition.
You can do a broadband CID, meaning you just fragment all the charge states. And you can do a narrow band MS/MS, meaning you select a few charge state for fragmentation. And the last one is wide-- I'm sorry.
Narrow quad is select one charge states, and the wide quad is select few charge state mass window for top down. So any one of those works really well. And here, just to show you the mass accuracy from the fragmentation, those are terminal fragment ions. And I just want to show example of a B86 and a B131. And overall, you get less than 500 ppb mass accuracy consistently.
OK? So that's pretty impressive for top-down fragmentation. And we sent the data to put the data into this clip MS software for sequence confirmation for top-down experiment. And overall, for the carbonic anhydrase, we got 51% sequence coverage from the experiment. And then here, I just want to highlight the power of high mass resolution.
There is a fragment ion in the data that's the internal fragmentation coming from B41 and the Y132. So it's in the middle. And this fragment ion has a mass accuracy at 193 parts per billion. And it match-- well, I think it's offline slightly.
But it match well with the periodic predictor model. And if you look at the possible candidate for that mass, for that internal fragmentation, there's two others also very close to the theoretical predicted fragment. It's less than 1 dalton. But only this one gave you the best mass accuracy. So the other two are outside the search tolerance that we set at 750 ppb. So this 750 ppb is being rigorously tested, and it's a very reliable mass tolerance window for doing top-down fermentation assignment.
The last one, I just want to show that the MRT system is also capable of doing native mass spec data. I don't have a lot of data on that. We just start collecting data. But just to show you a couple of examples, the top one is carbonic anhydrase. I think it's about 40k-ish. This, you can collect the data in the protein mode.
You definitely get isotope-resolved spectrum. And the resolution was measured at 210,000. And then, the B, C, and D, that's NIST map, ADH tetramer, and the aldolase tetramer. All three of them has very similar molecular weight. It's about 150k. And you can see that under the native MS condition, you do push the charged state to lower charge state at the higher mass.
But the data quality looks very good. If you just zoom into one of the charge state in this map data, then you can clearly see the different forms of glycan. And by the way, when we collect the data for native mass spec, you can extend the mass range all the way to 20,000 AMU.
OK? So in summary-- and right on time-- I hope that I show you the design and performance of this new, novel hybrid time-of-flight Q-Tof instrument. And then it has really long flight paths with minimum signal loss, and it gives you super high mass resolution and the mass accuracy. And then it has also fast scan speed. And also, what's the implication for biopharm application? I demonstrate some examples today.
You can select MRT mode, MRT protein mode, or diamond mode for a variety of applications. It's really depend on the size of your molecule. And I illustrated the subunit data using phabricator digestion and the reduced light chain heavy chain, subunit analysis, peptide mapping, intact mass, and native MS, and the top-down sequencing. So I hope there will be more work done on this instrument.
And then, this instrument will serve as the platform for the next generation of high-res mass spec instrument from Waters. So just expect more from Waters, and keep your eyes open. With that, I would like to acknowledge my colleague from UK Winslow's site, Dale Cooper-Shepherd, and also, my colleague from US Demo Lab Brad Williams, Barbara Sullivan. And James Langridge is the head of the advanced mass spec technology group; and also, Professor Joseph Loo for the native and top-down work that I showed today. And thank you for your time. [APPLAUSE] [INAUDIBLE],, are we suppose to ask question? Do you have any questions? Or are we stay for questions? Yeah, if you have a question, please.
Yeah, you talked a bit about the different ionization modes. How much is required to switch in between those ionization modes? Is there removal of the front end and some reconfiguring? Oh, you mean the MRT? No, you just do it in the Method setting. You don't have to change anything. No, I'm talking about the source. You mentioned that it has MALDI capability. Oh, the MALDI, DESI, and-- yes, you do have to switch those.
I have a quick question also. In the, let's say, peptide map mode, what is the typical acquisition rate if one wants to do data dependent? I think we usually use 1 or 2 hertz. One to two? Yeah. OK, thank you. If you have more questions, just stick around. I'll be in the poster session.
You know what I look like. All right, thank you again. [APPLAUSE]
2024-02-04 01:44